HKUST-1
Als HKUST-1 (HKUST ⇒ Hong Kong University of Science and Technology),[1] auch bekannt als MOF-199,[2] wird eine Strukturfamilie bezeichnet, die zu der Gruppe der Metall-organischen Gerüstverbindungen gehören.
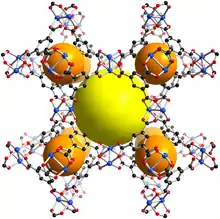
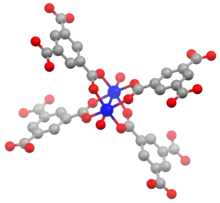
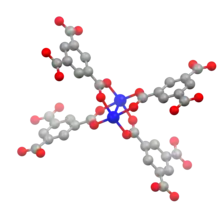
Beschreibung
Metall-organische Gerüstverbindungen sind kristalline Materialien, in welchen Metallzentren durch Brückenliganden (sogenannte Linker) dreidimensional in sich wiederholenden Koordinationseinheiten verbunden sind. Die HKUST-1-Struktur wird von dimeren Metallzentren aufgebaut, welche durch Benzol-1,3,5-tricarboxylat-Linkermoleküle verbunden sind. Eine dimere Metalleinheit, an die vier Benzol-1,3,5-tricarboxylatlinker gebunden sind, wird als Schaufelrad bezeichnet und wird häufig als sekundäre Baueinheit (englisch secondary building unit, SBU) der HKUST-1-Struktur bezeichnet. Im hydratisierten Zustand, welcher üblicherweise vorliegt wenn HKUST-1-Materialien an Luft aufbewahrt werden, ist ein Wassermolekül an jedes der zwei Metallzentren an der axialen Position der SBU koordiniert. Durch einen Aktivierungsprozess bei erhöhten Temperaturen oder unter Vakuum können diese Wassermoleküle entfernt werden, wodurch der desolvatisierte Zustand erreicht wird, in dem ein Koordinationsplatz an jedem Metallzentrum unbesetzt ist. Diese freie Koordinationsstelle wird als koordnatives ungesättigtes Zentrum (englisch coordinatively unsaturated site, CUS) bezeichnet und steht für direkte Metall-Substrat-Wechselwirkungen mit Gastmoleküle in den Poren zur Verfügung. Im desolvatisierten Zustand ist die Summenformel von HKUST-1-Materialien M3(BTC)2.
Strukturelle Analoga
Monometallische HKUST-1-Analoga
Cu2+ wurde als Metallzentrum in dem ersten entwickelten HKUST-1-Material verwendet.[1] Inzwischen wurde die HKUST-1-Struktur auch mit anderen Metallen hergestellt, die überwiegend einen Oxidationszustand von +2 besitzen. Dabei ist die gesamte Gerüststruktur neutral. Werden jedoch trivalente Metalle (Oxidationszustand +3) eingesetzt, so ist die Gerüststruktur positiv geladen und es benötigt Anionen um diese Ladung zu kompensieren und die Ladungsneutralität zu gewährleisten. Die Anionen sind dann statt Lösungsmittelmolekülen an den axialen Positionen der Schaufelradeinheiten und können nicht ohne weiteres entfernt werden.[3]
Metallzentrum und Oxidationszahl |
Jahr der Erstveröffentlichung |
Alternativer Name |
Zitation |
---|---|---|---|
Cu2+ | 1999 | Cu3BTC2, CuBTC |
[1][4] |
Mo2+ | 2006 | TUDMOF-1 | [5] |
Fe2+/3+ | 2007 | - | [6] |
Cr2+ | 2010 | - | [7] |
Ni2+ | 2011 | - | [8] |
Zn2+ | 2011 | - | [9] |
Ru2+/3+ | 2011 | - | [10] |
Co2+ | 2012 | - | [11] |
Fe2+ | 2012 | - | [11] |
Mn2+ | 2012 | - | [11] |
Fe3+ | 2014 | - | [3] |
Ru2+ | 2016 | - | [12] |
Multimetallische HKUST-1-Analoga
Zusätzlich zu monometallischen HKUST-1-Materialien wurden verschiedene multimetallische HKUST-1-Analoga hergestellt, welche zwei oder mehr Metalle in ihrer Gerüststruktur enthalten. Diese Metalle sind über äquivalente Positionen im Gerüst verteilt und die Metallverhältnisse können häufig beliebig variiert werden. Der Einbau von mehreren Metallen in eine HKUST-1-Struktur kann durch eine direkte Synthese oder durch einen post-synthetischen Metallaustausch erreicht werden. Während bei der direkten Synthese alle Metalle in einem Syntheseschritt eingebaut werden wird bei einem post-synthetischen Metallaustausch zunächst ein monometallischen HKUST-1-Material hergestellt, welches in einem zweiten Schritt in einer Metallsalzlösung eines zweiten Metalles suspendiert wird. Dabei wird ein Teil der Metallzentren der monometallischen HKUST-1-Struktur durch das neue Metall ersetzt und somit eine bimetallische HKUST-1-Struktur erhalten.
Metallzentren und Oxidationszahlen |
Synthesemethode | Zitation |
---|---|---|
Cu2+ / Zn2+ | Direkte Synthese | [13][14] |
Direkte Synthese
Kugelmühle (mechanochemical) |
[15] | |
Cu2+ / Fe2+ | Post-synthetischer Metallaustausch | [16] |
Cu2+ / Co2+ | Post-synthetischer Metallaustausch | [16] |
Cu2+ / Ni2+ | Direkte Synthese | [17] |
Cu2+ / Pd2+ | Direkte Synthese | [18][19] |
Cu2+ / Ru3+ | Direkte Synthese | [20] |
Ru2+/3+ / Rh2+ | Direkte Synthese | [21] |
Cu2+ / Ag+ | Post-synthetischer Metallaustausch | [22] |
Cu2+ / Fe3+ | Direkte Synthese | [23] |
Cu2+ / Mn2+ | Post-synthetischer Metallaustausch | [16] |
Cu2+ / Zn2+ / Mo6+ | Direkte Synthese
Kugelmühle (mechanochemical) |
[15] |
Theoretisch berechnete HKUST-1-Analoga
Viele HKUST-1-Materialien wurden bereits hergestellt und erforsch. Darüber hinaus wurden die Materialeigenschaften der HKUST-1-Struktur auch mithilfe von theoretischen Berechnungen untersucht. Zu diesem Zweck wurden auch HKUST-1-Analoga angenommen, die so bislang nicht synthetisiert wurden. Dazu zählen sowohl monometallische HKUST-1 mit den Metallen Scandium, Vanadium, Titan, Wolfram oder Cadmium,[24][25] als auch bimetallische HKUST-1, welche mit Kupfer in Kombination mit einem zweiten Metall (Wolfram, Rhenium, Osmium, Iridium, Platin, Gold) enthalten.[26][27]
Eigenschaften
Die HKUST-1-Struktur ist empfindlich gegenüber Wasser und Luftfeuchtigkeit. Bei längerem Kontakt führt dies zu einer Zersetzung der Gerüststruktur, wobei die Zeitspanne stark variiert (Minuten bis Wochen) und von dem Metallzentrum abhängt.
Einzelnachweise
- S. S. Chui: A Chemically Functionalizable Nanoporous Material [Cu3(TMA)2(H2O)3]n. In: Science. Band 283, Nr. 5405, 19. Februar 1999, S. 1148–1150, doi:10.1126/science.283.5405.1148.
- D. Britt, D. Tranchemontagne, O. M. Yaghi: Metal-organic frameworks with high capacity and selectivity for harmful gases. In: Proceedings of the National Academy of Sciences. Band 105, Nr. 33, 19. August 2008, ISSN 0027-8424, S. 11623–11627, doi:10.1073/pnas.0804900105.
- S. A. Sotnik, S. V. Kolotilov, M. A. Kiskin, Zh. V. Dobrokhotova, K. S. Gavrilenko: Synthesis, crystal structure, and physicochemical properties of the new metal-organic framework — the iron(iii) complex with benzene-1,3,5-tricarboxylate. In: Russian Chemical Bulletin. Band 63, Nr. 4, April 2014, ISSN 1066-5285, S. 862–869, doi:10.1007/s11172-014-0522-x.
- Qing Min Wang, Dongmin Shen, Martin Bülow, Miu Ling Lau, Shuguang Deng: Metallo-organic molecular sieve for gas separation and purification. In: Microporous and Mesoporous Materials. Band 55, Nr. 2, September 2002, S. 217–230, doi:10.1016/S1387-1811(02)00405-5.
- Markus Kramer, Ulrich Schwarz, Stefan Kaskel: Synthesis and properties of the metal-organic framework Mo3(BTC)2 (TUDMOF-1). In: Journal of Materials Chemistry. Band 16, Nr. 23, 2006, ISSN 0959-9428, S. 2245, doi:10.1039/b601811d.
- Linhua Xie, Shuxia Liu, Chaoying Gao, Ruige Cao, Jianfang Cao: Mixed-Valence Iron(II, III) Trimesates with Open Frameworks Modulated by Solvents. In: Inorganic Chemistry. Band 46, Nr. 19, September 2007, ISSN 0020-1669, S. 7782–7788, doi:10.1021/ic062273m.
- Leslie J. Murray, Mircea Dinca, Junko Yano, Sachin Chavan, Silvia Bordiga: Highly-Selective and Reversible O2 Binding in Cr3(1,3,5-benzenetricarboxylate)2. In: Journal of the American Chemical Society. Band 132, Nr. 23, 16. Juni 2010, ISSN 0002-7863, S. 7856–7857, doi:10.1021/ja1027925.
- Palanikumar Maniam, Norbert Stock: Investigation of Porous Ni-Based Metal–Organic Frameworks Containing Paddle-Wheel Type Inorganic Building Units via High-Throughput Methods. In: Inorganic Chemistry. Band 50, Nr. 11, 6. Juni 2011, ISSN 0020-1669, S. 5085–5097, doi:10.1021/ic200381f.
- Jeremy I. Feldblyum, Ming Liu, David W. Gidley, Adam J. Matzger: Reconciling the Discrepancies between Crystallographic Porosity and Guest Access As Exemplified by Zn-HKUST-1. In: Journal of the American Chemical Society. Band 133, Nr. 45, 16. November 2011, ISSN 0002-7863, S. 18257–18263, doi:10.1021/ja2055935.
- Olesia Kozachuk, Kirill Yusenko, Heshmat Noei, Yuemin Wang, Stephan Walleck: Solvothermal growth of a ruthenium metal–organic framework featuring HKUST-1 structure type as thin films on oxide surfaces. In: Chemical Communications. Band 47, Nr. 30, 2011, ISSN 1359-7345, S. 8509, doi:10.1039/c1cc11107h.
- Zhenjie Zhang, Linping Zhang, Lukasz Wojtas, Mohamed Eddaoudi, Michael J. Zaworotko: Template-Directed Synthesis of Nets Based upon Octahemioctahedral Cages That Encapsulate Catalytically Active Metalloporphyrins. In: Journal of the American Chemical Society. Band 134, Nr. 2, 18. Januar 2012, ISSN 0002-7863, S. 928–933, doi:10.1021/ja208256u.
- Wenhua Zhang, Kerstin Freitag, Suttipong Wannapaiboon, Christian Schneider, Konstantin Epp: Elaboration of a Highly Porous Ru II,II Analogue of HKUST-1. In: Inorganic Chemistry. Band 55, Nr. 24, 19. Dezember 2016, ISSN 0020-1669, S. 12492–12495, doi:10.1021/acs.inorgchem.6b02038.
- Bettina Jee, Konrad Eisinger, Farhana Gul-E-Noor, Marko Bertmer, Martin Hartmann: Continuous Wave and Pulsed Electron Spin Resonance Spectroscopy of Paramagnetic Framework Cupric Ions in the Zn(II) Doped Porous Coordination Polymer Cu3-xZnx(btc)2. In: The Journal of Physical Chemistry C. Band 114, Nr. 39, 7. Oktober 2010, ISSN 1932-7447, S. 16630–16639, doi:10.1021/jp105955w.
- Farhana Gul-E-Noor, Bettina Jee, Matthias Mendt, Dieter Himsl, Andreas Pöppl: Formation of Mixed Metal Cu3-xZnx(btc)2 Frameworks with Different Zinc Contents: Incorporation of Zn2+ into the Metal–Organic Framework Structure as Studied by Solid-State NMR. In: The Journal of Physical Chemistry C. Band 116, Nr. 39, 4. Oktober 2012, ISSN 1932-7447, S. 20866–20873, doi:10.1021/jp3054857.
- Su-Kyung Lee, Do-Young Hong, Myung-Geun Jeong, Ji Woong Yoon, Jongyoon Bae: Trimetallic copper trimesate with isomorphously substituted Mo(VI) and its catalytic properties. In: Microporous and Mesoporous Materials. Band 253, November 2017, S. 223–232, doi:10.1016/j.micromeso.2017.07.007.
- Dorina F. Sava Gallis, Marie V. Parkes, Jeffery A. Greathouse, Xiaoyi Zhang, Tina M. Nenoff: Enhanced O2 Selectivity versus N2 by Partial Metal Substitution in Cu-BTC. In: Chemistry of Materials. Band 27, Nr. 6, 24. März 2015, ISSN 0897-4756, S. 2018–2025, doi:10.1021/cm5042293.
- Jue Hu, Huijing Yu, Wei Dai, Xiaoyang Yan, Xin Hu: Enhanced adsorptive removal of hazardous anionic dye “congo red” by a Ni/Cu mixed-component metal–organic porous material. In: RSC Adv. Band 4, Nr. 66, 2014, ISSN 2046-2069, S. 35124–35130, doi:10.1039/C4RA05772D.
- Wenhua Zhang, Zhihao Chen, Majd Al-Naji, Penghu Guo, Stefan Cwik: Simultaneous introduction of various palladium active sites into MOF via one-pot synthesis: Pd@[Cu3-xPdx(BTC)2]n. In: Dalton Transactions. Band 45, Nr. 38, 2016, ISSN 1477-9226, S. 14883–14887, doi:10.1039/C6DT02893D.
- Penghu Guo, Christian Froese, Qi Fu, Yen-Ting Chen, Baoxiang Peng: CuPd Mixed-Metal HKUST-1 as a Catalyst for Aerobic Alcohol Oxidation. In: The Journal of Physical Chemistry C. Band 122, Nr. 37, 20. September 2018, ISSN 1932-7447, S. 21433–21440, doi:10.1021/acs.jpcc.8b05882.
- Meike A. Gotthardt, Roland Schoch, Silke Wolf, Matthias Bauer, Wolfgang Kleist: Synthesis and characterization of bimetallic metal–organic framework Cu–Ru-BTC with HKUST-1 structure. In: Dalton Transactions. Band 44, Nr. 5, 2015, ISSN 1477-9226, S. 2052–2056, doi:10.1039/C4DT02491E.
- Werner R. Heinz, Tim Kratky, Markus Drees, Andreas Wimmer, Ondřej Tomanec: Mixed precious-group metal–organic frameworks: a case study of the HKUST-1 analogue [RuxRh3-x(BTC)2]. In: Dalton Transactions. Band 48, Nr. 32, 2019, ISSN 1477-9226, S. 12031–12039, doi:10.1039/C9DT01198F.
- Zhiguo Sun, Gang Li, Yue Zhang, Hai-ou Liu, Xionghou Gao: Ag-Cu-BTC prepared by postsynthetic exchange as effective catalyst for selective oxidation of toluene to benzaldehyde. In: Catalysis Communications. Band 59, Januar 2015, S. 92–96, doi:10.1016/j.catcom.2014.09.047.
- Johannes Bitzer, Steffen Otterbach, Kavipriya Thangavel, Anastasia Kultaeva, Rochus Schmid: Experimental Evidence for the Incorporation of Two Metals at Equivalent Lattice Positions in Mixed‐Metal Metal–Organic Frameworks. In: Chemistry – A European Journal. Band 26, Nr. 25, 4. Mai 2020, ISSN 0947-6539, S. 5667–5675, doi:10.1002/chem.201905596.
- Marie V. Parkes, Dorina F. Sava Gallis, Jeffery A. Greathouse, Tina M. Nenoff: Effect of Metal in M3(btc)2 and M2(dobdc) MOFs for O2/N2 Separations: A Combined Density Functional Theory and Experimental Study. In: The Journal of Physical Chemistry C. Band 119, Nr. 12, 26. März 2015, ISSN 1932-7447, S. 6556–6567, doi:10.1021/jp511789g.
- Tian-ding Hu, Yan Jiang, Yi-hong Ding: Computational screening of metal-substituted HKUST-1 catalysts for chemical fixation of carbon dioxide into epoxides. In: Journal of Materials Chemistry A. Band 7, Nr. 24, 2019, ISSN 2050-7488, S. 14825–14834, doi:10.1039/C9TA02455G.
- Qiuju Zhang, Lujie Cao, Baihai Li, Liang Chen: Catalyzed activation of CO2 by a Lewis-base site in W-Cu-BTC hybrid metal organic frameworks. In: Chemical Science. Band 3, Nr. 9, 2012, ISSN 2041-6520, S. 2708, doi:10.1039/c2sc20521a.
- Xiuqin Dong, Xiuyu Liu, Yifei Chen, Minhua Zhang: Screening of bimetallic M-Cu-BTC MOFs for CO2 activation and mechanistic study of CO2 hydrogenation to formic acid: A DFT study. In: Journal of CO2 Utilization. Band 24, März 2018, S. 64–72, doi:10.1016/j.jcou.2017.11.014.