Phosphoramidit-Synthese
Die Phosphoramidit-Synthese ist eine Methode der Biochemie zur Herstellung von RNA- oder DNA-Sequenzen aus Nukleosid-Phosphoramiditen.[1] Sie ist eine Methode zur künstlichen DNA-Synthese, die Produkte gehören zur synthetischen DNA.
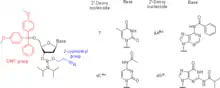
Prinzip
Phosphoramidite werden als Nukleotidanaloga zur Synthese von Oligonukleotiden verwendet. Zur Vermeidung von Nebenreaktionen an anderen reaktiven nucleophilen Gruppen (z. B. Hydroxy- oder Aminogruppen) werden diese mit Schutzgruppen versehen. Zwei nucleophile Gruppen (meist zwei Hydroxygruppen) werden nicht geschützt, sondern zur Erzeugung des Phosphoramidits verwendet. Per Phosphoramidit-Synthese können Nukleinsäuren, Nukleinsäure-Analoga, LNA, Morpholino, Nukleotide mit Modifikationen an der 2′-Position (z. B. Methoxy-geschützte Amine, Fluoride), Nukleotide mit nicht natürlichen Nukleinbasen (z. B. Hypoxanthin, Xanthin), trizyklische Nukleinbasen wie die G-clamp,[2] selektiv reaktive Gruppen oder fluoreszente Nukleinbasederivate erzeugt werden.
Herstellung der Phosphoramidite
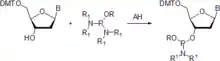
Phosphoramidite werden über drei Methoden erzeugt. Meist wird die freie Hydroxygruppe mit Phosphorodiamidit in Anwesenheit einer schwachen Säure aktiviert.[3][4] Da manche Bisamidite nicht thermostabil sind,[5] wird meistens 2-Cyanoethyl-N,N,N′,N′-tetraisopropylphosphorodiamidit verwendet, welches in zwei Arbeitsschritten und einer Vakuumdestillation hergestellt werden kann.[6][7]
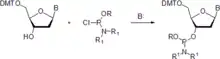
Phosphoramidite mit Schutzgruppen können auch mit Phosphorchloridit in Anwesenheit einer organischen Base erzeugt werden, meist N,N-Diisopropylethylamin (Hünigsche Base).[8]

Als dritte Methode werden die geschützten Nukleinbasen zuerst mit Chlor-N,N,N′,N′-tetraisopropylphosphordiamidit in Anwesenheit einer organischen Base wie die Hunigsche Base behandelt, um ein geschütztes Nukleosid-Phosphordiamidit zu erhalten. Anschließend erfolgt die Zugabe eines der Phosphitschutzgruppe entsprechenden Alkohols (z. B. 2-Cyanoethanol) und einer schwachen Säure.[9]
Die erzeugten Nucleosid-Phosphoramidite werden durch Kieselgel-Säulenchromatographie gereinigt. Zur Erhaltung der Stabilität der Phosphoramidite wird zwischen drei und fünf Prozent Triethylamin im Puffer verwendet. Die Reinheit kann unter anderem durch 31P NMR-Spektroskopie ermittelt werden. Das Chiralitätszentrum am P(III)-Atom weist zwei Maxima bei etwa 149 ppm auf, entsprechend den beiden Diastereomeren. Unerwünschte Phosphit-Triester besitzen eine Verschiebung von 138 bis 140 ppm und H-Phosphonate besitzen zwei Maxima bei 8 und 10 ppm.
Eigenschaften der Phosphoramidite
Nucleosid-Phosphoramidite sind in wasser- und sauerstofffreier Pulverform bei 4 °C und in basischen Lösungen einigermaßen stabil. Sie zerfallen jedoch unter sauren Bedingungen. Die Halbwertszeit von 2-Cyanoethyl-5′-O-(4,4′-Dimethoxytrityl)Thymidin-3′-O-(N,N-diisopropylamino)phosphit in 95 % wässrigem Acetonitril bei 25 °C liegt bei 200 h.[10]

Die wichtigste Eigenschaft der Phosphoramidite ist die bereitwillige und schnelle Reaktion mit Nukleophilen in Anwesenheit von Azol-Katalysatoren, wie 1H-Tetrazol, 2-Ethylthiotetrazol,[11] 2-Benzylthiotetrazol,[12][13] 4,5-Dicyanoimidazol,[14] oder ähnlichen Stoffen. Die Kopplung der Phosphoramidite führt zu einer Epimerisierung am Chiralitätszentrum. Wenn Wasser als Nukleophil verwendet wird, ist das Produkt ein H-Phosphonatdiester, weshalb eine Reaktion mit Wasser eine häufige unerwünschte Nebenreaktion bei der Synthese von Oligonukleotiden darstellt.
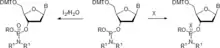
Phosphoramidite werden leicht zu Phosphoramidaten oxidiert, z. B. mit wässrigem Iod in Anwesenheit schwacher Basen oder mit Wasserstoffperoxid.[15] Weiterhin reagieren Phosphoramidite mit Chalkogenen. Bei Kontakt mit Schwefel-haltigen Stoffen werden Phosphorthioamidate gebildet.[15][16][17][18] Die Reaktion mit Selen führt zu Phosphorselenoamidaten.[15][16][19] Hierbei wird die Konfiguration erhalten.
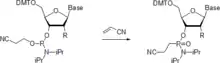
Nucleosid-Phosphoramidite werden durch die Michaelis-Arbusow-Reaktion in Phosphonamidate umgewandelt.[20] Die Reaktion ist bei Raumtemperatur stereoselektiv unter Erhalt der Konfiguration. Bei 55 °C erfolgt die Racemisierung.
Ähnlich wie bei Phosphinen und tertiären Phosphiten kann eine Staudinger-Reaktion erfolgen.
- (RO)2P-N(R1)2 + R2-N3 + H2O → (RO)2P(=O)-N(R1)2 + R2-NH2 + N2
Schutzgruppen
Die natürlich vorkommenden Nukleotide (Nucleosid-3′- oder Nucleosid-5′-Phosphate) und ihre Phosphodiester-Analoga sind zu wenig reaktiv für eine hohe Ausbeute. Durch 3′-O-(N,N-diisopropyl phosphoramidite)-Derivate wird die Bindungsbildung bei der Phosphit-Triester-Methode deutlich beschleunigt. Zur Vermeidung unerwünschter Nebenreaktionen werden alle nicht zur Kopplung benötigten reaktiven Gruppen mit Schutzgruppen versehen. Nach Fertigstellung der DNA-Sequenz werden die Schutzgruppen entfernt.[21][22][23][24][25] Phosphoramidite sind oftmals an der 5′-Hydroxygruppe mit 4,4′-Dimethoxytrityl geschützt. Thymin and Uracil besitzen keine freien Aminogruppen und benötigen daher keine Schutzgruppen. Dagegen werden Adenin, Cytosin und Guanin an der Aminogruppe geschützt, da diese sonst reaktiv ist. Obwohl diese Nukleinbasen auch ungeschützt eingesetzt werden können,[26] werden meistens Basen-labile Schutzgruppen verwendet und bis zum letzten Zyklus beibehalten.
Zwei verschiedene Schutzgruppentypen werden bei der Phosphoramidit-Synthese verwendet. Der erste Typ umfasst Benzoyl-Schutzgruppen am N4-Atom der Cytosine und am N6-Atom der Adenine (A, dA, C, dC). Guanine (G und dG) werden mit einer Isobutyrylgruppe geschützt. Cytosine können auch durch eine Acetylgruppe geschützt werden.[27] Der zweite Schutzgruppentyp umfasst Isobutyrylgruppen an Adeninen (A und dA)[28] oder Phenoxyacetylgruppen (PAC).[29] Cytosine tragen eine Acetylgruppe[27] und Guanine werden mit 4-Isopropylphenoxyacetylgruppen (i-Pr-PAC)[30] oder Dimethylformamidinogruppen (dmf) geschützt.[31] Schutzgruppen des zweiten Typs lassen sich schneller abspalten, sind im Allgemeinen aber weniger stabil in Lösungen.
Die Phosphitgruppe wird durch eine basenlabile 2-Cyanoethylgruppe geschützt.[32] Nach der Bindung des Phosphoramidits an das wachsende Oligonukleotid und der Konversion des Phosphitanteils zum P(V) ist die Anwesenheit der Phosphatschutzgruppe nicht notwendig für weitere Kopplungsschritte.[33]
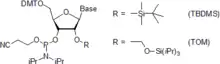
Bei der RNA-Synthese werden die 2′-Hydroxygruppen mit t-Butyldimethylsilylgruppen (TBDMS)[34][35][36][37] oder mit Tri-iso-propylsilyloxymethylgruppen (TOM) geschützt.[38][39] Beide Schutzgruppen sind mit Fluoriden entfernbar.
Der Phosphitanteil trägt auch eine Diisopropylaminogruppe (iPr2N), die unter sauren Bedingungen reaktiv ist. Nach der Aktivierung ist die Diisopropylaminogruppe eine Abgangsgruppe bei der Bindung an das wachsende Oligonukleotid.
Meistens wird das Reaktionsprodukt nach der Synthese gereinigt, z. B. per Fällung oder per Hochleistungsflüssigkeitschromatographie. Durch Verwendung eines polymerisierbaren Moleküls (mit Methacrylsäure-Gruppe) im letzten Kopplungsschritt der Phosphoramiditsynthese können nach einer anschließenden Polymerisation die korrekt synthetisierten Oligonukleotide von den fehlerhaften und acetylierten Kopplungsprodukten abgetrennt werden.[40]
Festphasensynthese
Nukleinsäuren können durch Festphasensynthese mittels Phosphoramiditen dargestellt werden. Als Trägermaterial kann CPG (controlled pore glass) verwendet werden. Die Nukleinsäure wird mithilfe eines Kupplungsreagenz an die Oberfläche der Festphase kondensiert. Die Kupplung der Nukleinsäuren erfolgt durch Umsetzung der ankondensierten Nukleinsäure mit einem Phosphoramidit-Derivat der anderen Nukleinsäure. Um die Abtrennung von nicht umgesetzten Nukleinsäuren zu erleichtern, wird die freie Alkoholgruppe der Festphase acetyliert. Dieser Vorgang wird als Capping bezeichnet. Nachfolgend wird der Phosphor oxidiert. Die Nukleinsäure kann entweder aufgereinigt oder in einem weiteren Cyclus weiter umgesetzt werden.
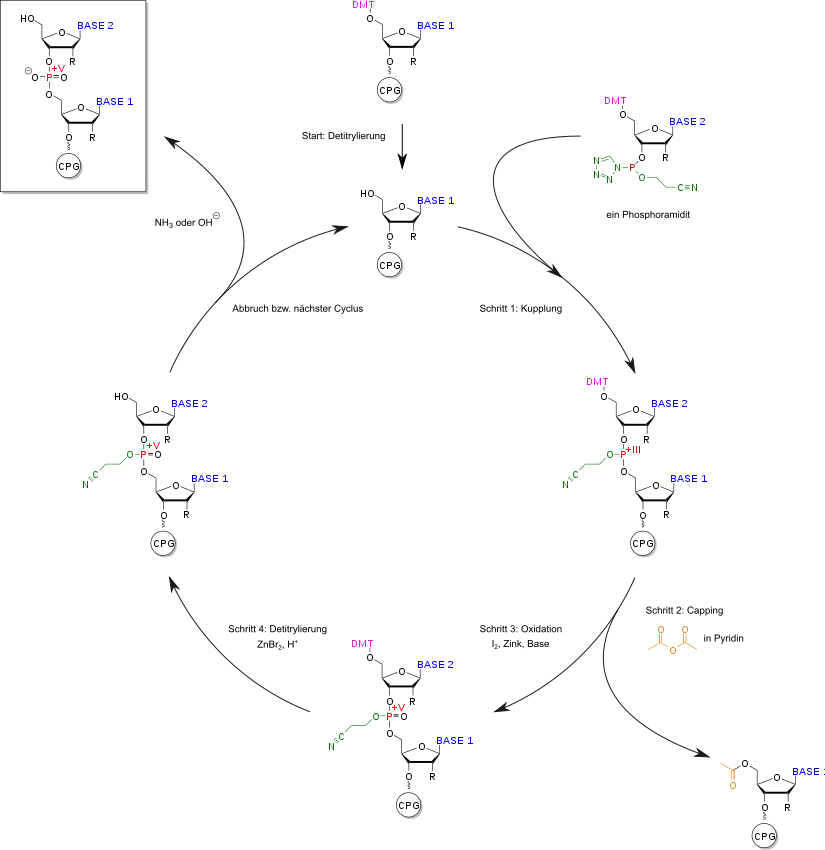
Literatur
- Comprehensive Natural Products Chemistry, Volume 7: DNA and Aspects of Molecular Biology. Kool, Eric T.; Editor. Neth. (1999), 733 pp. Publisher: (Elsevier, Amsterdam, Neth.)
- Beaucage, S. L.; Iyer, R. P. Advances in the synthesis of oligonucleotides by the phosphoramidite approach. Tetrahedron 1992, 48, 2223–2311.
- Beaucage, S. L.; Iyer, R. P. The functionalization of oligonucleotides via phosphoramidite derivatives. Tetrahedron 1993, 49, 1925–1963.
- Beaucage, S. L.; Iyer, R. P. The synthesis of modified oligonucleotides by the phosphoramidite approach and their applications. Tetrahedron 1993, 49, 6123–6194.
- Beaucage, S L. Oligodeoxyribonucleotides synthesis. Phosphoramidite approach. Methods in Molecular Biology (Totowa, NJ, United States) (1993), 20 (Protocols for Oligonucleotides and Analogs), 33–61.
- Reese, C. B. The chemical synthesis of oligo- and poly-nucleotides: a personal commentary. Tetrahedron 2002, 58, 8893–8920.
- Brown T., Brown D. J. S. 1991. In Oligonucleotides and Analogues. A Practical Approach, ed. F Eckstein, pp. 1 – 24. Oxford: IRL
Einzelnachweise
- Beaucage, S.L.; Marvin H. Caruthers: Deoxynucleoside phosphoramidites—A new class of key intermediates for deoxypolynucleotide synthesis. In: Tetrahedron Letters. 22, 1981, S. 1859–1862. doi:10.1016/S0040-4039(01)90461-7.
- Lin, K.-Y., Matteucci, M. D.: A cytosine analog capable of clamp-like binding to a guanine in helical nucleic acids. In: J. Amer. Chem. Soc.. 120, Nr. 33, 1998, S. 8531–8532. doi:10.1021/ja981286z.
- Nielsen, J.; Marugg, J. E.; Taagaard, M.; Van Boom, J. H.; Dahl, O.: Polymer-supported synthesis of deoxyoligonucleotides using in situ prepared deoxynucleoside 2-cyanoethyl phosphoramidites. In: Rec. Trav. Chim. Pays-Bas. 105, Nr. 1, 1986, S. 33–34.
- Nielsen, J.; Taagaard, M.; Marugg, J. E.; Van Boom, J. H.; Dahl, O.: Application of 2-cyanoethyl N,N,N′,N′-tetraisopropylphosphorodiamidite for in situ preparation of deoxyribonucleoside phosphoramidites and their use in polymer-supported synthesis of oligodeoxyribonucleotides. In: Nucl. Acids Res.. 14, Nr. 18, 1986, S. 7391–7403. doi:10.1093/nar/14.18.7391.
- Nielsen, J.; Marugg, J. E.; Van Boom, J. H.; Honnens, J.; Taagaard, M.; Dahl, O.: Thermal instability of some alkyl phosphorodiamidites. In: J. Chem Res. Synopses. Nr. 1, 1986, S. 26–27.
- Nielsen, J.; Dahl, O.: Improved synthesis of 2-cyanoethyl N,N,N′,N′-tetraisopropylphosphorodiamidite (iPr2N)2POCH2CH2CN. In: Nucl. Acids Res.. 15, Nr. 8, 1987, S. 3626. doi:10.1093/nar/15.8.3626.
- Beaucage, S. L.: 2-Cyanoethyl Tetraisopropylphosphorodiamidite. In: e-EROS Encyclopedia of Reagents for Organic Synthesis. 2001. doi:10.1002/047084289X.rn00312.
- Sinha, N. D.; Biernat, J.; Koester, H.: β-Cyanoethyl N,N-dialkylamino/N-morpholinomonochloro phosphoamidites, new phosphitylating agents facilitating ease of deprotection and work-up of synthesized oligonucleotides. In: Tetrahedron Lett.. 24, Nr. 52, 1983, S. 5843–5846. doi:10.1016/S0040-4039(00)94216-3.
- Marugg, J. E.; Burik, A.; Tromp, M.; Van der Marel, G. A.; Van Boom, J. H.: A new and versatile approach to the preparation of valuable deoxynucleoside 3′-phosphite intermediates. In: Tetrahedron Lett.. 24, Nr. 20, 1986, S. 2271–22274. doi:10.1016/S0040-4039(00)84506-2.
- Guzaev, A. P.; Manoharan, M.: 2-Benzamidoethyl group - a novel type of phosphate protecting group for oligonucleotide synthesis. In: J. Amer. Chem. Soc.. 123, Nr. 5, 2001, S. 783–793. doi:10.1021/ja0016396.
- Sproat, B.; Colonna, F.; Mullah, B.; Tsou, D.; Andrus, A.; Hampel, A.; Vinayak, R.: An efficient method for the isolation and purification of oligoribonucleotides. In: Nucleosides & Nucleotides. 14, Nr. 1&2, Februar 1995, ISSN 0261-3166, S. 255–273. doi:10.1080/15257779508014668.
- Stutz, A.; Hobartner, C.; Pitsch, S.: Novel fluoride-labile nucleobase-protecting groups for the synthesis of 3'(2')-O-amino-acylated RNA sequences. In: Helv. Chim. Acta. 83, Nr. 9, September 2000, ISSN 0018-019X, S. 2477–2503. doi:10.1002/1522-2675(20000906)83:9<2477::AID-HLCA2477>3.0.CO;2-9.
- Welz, R.; Muller, S.: 5-(Benzylmercapto)-1H-tetrazole as activator for 2′-O-TBDMS phosphoramidite building blocks in RNA synthesis. In: Tetrahedron Letters. 43, Nr. 5, Januar 2002, ISSN 0040-4039, S. 795–797. doi:10.1016/S0040-4039(01)02274-2.
- Vargeese, C.; Carter, J.; Yegge, J.; Krivjansky, S.; Settle, A.; Kropp, E.; Peterson, K.; Pieken, W.: Efficient activation of nucleoside phosphoramidites with 4,5-dicyanoimidazole during oligonucleotide synthesis. In: Nucl. Acids Res.. 26, Nr. 4, 1998, ISSN 0305-1048, S. 1046–1050. doi:10.1093/nar/26.4.1046. PMID 9461466. PMC 147346 (freier Volltext).
- Gacs-Baitz, E.; Sipos, F.; Egyed, O.; Sagi, G.: Synthesis and structural study of variously oxidized diastereomeric 5′-dimethoxytrityl-thymidine-3′-O-[O-(2-cyanoethyl)-N,N-diisopropyl]-phosphoramidite derivatives. Comparison of the effects of the P=O, P=S, and P=Se functions on the NMR spectral and chromatographic properties.. In: Chirality. 21, Nr. 7, 2009, S. 663–673.
- Nemer, M. J.; Ogilvie, K. K.: Phosphoramidate analogs of diribonucleoside monophosphates.. In: Tetrahedron Lett.. 21, Nr. 43, 1980, S. 4153–4154.
- Wilk, A.; Uznanski, B.; Stec, W. J.: Assignment of absolute configuration at phosphorus in dithymidylyl(3',5')phosphormorpholidates and -phosphormorpholidothioates.. In: Nucleosides & Nucleotides. 10, Nr. 1-3, 1991, S. 319–322.
- Guzaev, A. P.: Reactivity of 3H-1,2,4-dithiazole-3-thiones and 3H-1,2-dithiole-3-thiones as sulfurizing agents for oligonucleotide synthesis. In: Tetrahedron Letters. 52, 2011, S. 434–437. doi:10.1016/j.tetlet.2010.11.086.
- Holloway, G. A.; Pavot, C.; Scaringe, S. A.; Lu, Y.; Rauchfuss, T. B.: An organometallic route to oligonucleotides containing phosphoroselenoate.. In: ChemBioChem. 3, Nr. 11, 2002, S. 1061–1065.
- Ravikumar, V. T.; Kumar, R. K.: Stereoselective Synthesis of Alkylphosphonates: A Facile Rearrangement of Cyanoethyl-Protected Nucleoside Phosphoramidites. In: Org. Process Res. Dev.. 8, Nr. 4, 2004, S. 603–608. doi:10.1021/op030035u.
- Beta-Cyanoethyl Phosphoramidites. Products.appliedbiosystems.com. Abgerufen am 12. Mai 2009.
- Biosearch Technologies. Biosearchtech.com. Abgerufen am 12. Mai 2009.
- ChemGenes Corporation, a Biotechnology company. Chemgenes.com. Abgerufen am 12. Mai 2009.
- M. Powell: Applied Biosystems Instruments. Glenresearch.com. 17. Januar 2008. Abgerufen am 12. Mai 2009.
- Nucleic Acid Synthesis & Labeling. Thermo.com. 16. August 2008. Abgerufen am 12. Mai 2009.
- Gryaznov, S. M.; Letsinger, R. L.: Synthesis of oligonucleotides via monomers with unprotected bases. In: J. Amer. Chem. Soc.. 113, Nr. 15, 1991, S. 5876–5877. doi:10.1021/ja00015a059.
- Reddy, M. P.; Hanna, N. B.; Farooqui, F.: Ultrafast Cleavage and Deprotection of Oligonucleotides Synthesis and Use of CAc Derivatives. In: Nucleosides & Nucleotides. 16, 1997, S. 1589–1598. doi:10.1080/07328319708006236.
- McMinn, D.: Synthesis of oligonucleotides containing 3′-alkyl amines using N-isobutyryl protected deoxyadenosine phosphoramidite. In: Tetrahedron Lett.. 38, 1997, S. 3123. doi:10.1016/S0040-4039(97)00568-6.
- Schulhof, J. C.; Molko, D.; Teoule, R.: The final deprotection step in oligonucleotide synthesis is reduced to a mild and rapid ammonia treatment by using labile base-protecting groups. In: Nucleic Acids Res.. 15, Nr. 2, 1987, S. 397–416. doi:10.1093/nar/15.2.397. PMID 3822812. PMC 340442 (freier Volltext).
- Zhu, Q.: Observation and elimination of N-acetylation of oligonucleotides prepared using fast-deprotecting phosphoramidites and ultra-mild deprotection. In: Bioorg. & Med. Chem. Lett.. 11, 2001, S. 1105. doi:10.1016/S0960-894X(01)00161-5.
- McBride, L. J., R. Kierzek, S. L. Beaucage, M. H. Caruthers: Nucleotide chemistry. 16. Amidine protecting groups for oligonucleotide synthesis. In: J. Amer. Chem. Soc.. 108, 1986, S. 2040. doi:10.1021/ja00268a052.
- Sinha, N. D.; Biernat, J.; McManus, J.; Koester, H.: Polymer support oligonucleotide synthesis. XVIII: use of β-cyanoethyl-N,N-dialkylamino-/N-morpholino phosphoramidite of deoxynucleosides for the synthesis of DNA fragments simplifying deprotection and isolation of the final product. In: Nucleic Acids Res. 12, Nr. 11, 1984, S. 4539–4557. doi:10.1093/nar/12.11.4539. PMID 6547529. PMC 318857 (freier Volltext).
- Guzaev, A. P.; Manoharan, M.: Phosphoramidite Coupling to Oligonucleotides Bearing Unprotected Internucleosidic Phosphate Moieties. In: J. Org. Chem.. 66, Nr. 5, 2001, S. 1798–1804. doi:10.1021/jo001591e. PMID 11262130.
- Ogilvie, K. K.; Theriault, N.; Sadana, K. L.: Synthesis of oligoribonucleotides. In: J. Amer. Chem. Soc.. 99, Nr. 23, 1977, S. 7741–7743. doi:10.1021/ja00465a073.
- Usman, N.; Ogilvie, K. K.; Jiang, M. Y.; Cedergren, R. J.: The automated chemical synthesis of long oligoribuncleotides using 2′-O-silylated ribonucleoside 3′-O-phosphoramidites on a controlled-pore glass support: synthesis of a 43-nucleotide sequence similar to the 3′-half molecule of an Escherichia coli formylmethionine tRNA. In: J. Amer. Chem. Soc.. 109, Nr. 25, 1987, S. 7845–7854. doi:10.1021/ja00259a037.
- Usman, N.; Pon, R. T.; Ogilvie, K. K.: Preparation of ribonucleoside 3′-O-phosphoramidites and their application to the automated solid phase synthesis of oligonucleotides. In: Tetrahedron Lett.. 26, Nr. 38, 1985, S. 4567–4570. doi:10.1016/S0040-4039(00)98753-7.
- Scaringe, S. A.; Francklyn, C.; Usman, N.: Chemical synthesis of biologically active oligoribonucleotides using β-cyanoethyl protected ribonucleoside phosphoramidites. In: Nucl. Acids Res.. 18, Nr. 18, 1990, S. 5433–5441. doi:10.1093/nar/18.18.5433.
- Pitsch, S.; Weiss, P. A.; Wu, X.; Ackermann, D.; Honegger, T.: Fast and reliable automated synthesis of RNA and partially 2′-O-protected precursors ("caged RNA") based on two novel, orthogonal 2′-O-protecting groups. In: Helv. Chim. Acta. 82, Nr. 10, 1999, S. 1753–1761. doi:10.1002/(SICI)1522-2675(19991006)82:10<1753::AID-HLCA1753>3.0.CO;2-Y.
- Pitsch, S.; Weiss, P. A.; Jenny, L.; Stutz, A.; Wu, X.: Reliable chemical synthesis of oligoribonucleotides (RNA) with 2′-O-[(triisopropylsilyl)oxy]methyl(2′-O-tom)-protected phosphoramidites. In: Helv. Chim. Acta. 84, Nr. 12, 2001, S. 3773–3795. doi:10.1002/1522-2675(20011219)84:12<3773::AID-HLCA3773>3.0.CO;2-E.
- S. Fang, S. Fueangfung, Y. Yuan: Purification of synthetic oligonucleotides via catching by polymerization. In: Current protocols in nucleic acid chemistry / edited by Serge L. Beaucage ... [et al.]. Chapter 10Juni 2012, S. Unit10.14, ISSN 1934-9289. doi:10.1002/0471142700.nc1014s49. PMID 22700335.